Coanda Effect
Although generally unrecognized, Romanian aerodynamics pioneer Henri Coanda was actually the first person to build and fly a jet powered aircraft. It is commonly believed that the first jet engines were developed during World War II. Dr. Hans Von Ohain designed the first German jet aircraft, which made its first flight on August 27, 1939. Unaware of Dr. Von Ohain's work, A British engineer named Sir Frank Whittle also independently designed a jet aircraft, which first flew on May 15, 1941.
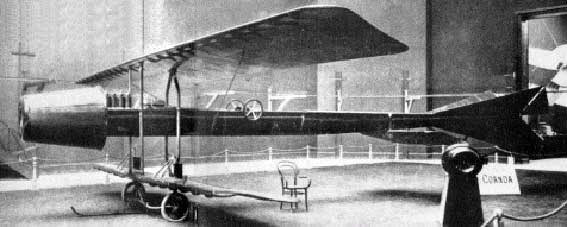
The Coanda- 1910, the world's first jet aircraft
Although these two men are generally thought of as the fathers of jet aircraft, Henri Coanda built and "flew" the first recorded jet aircraft about 30 years earlier.
In 1934 he obtained a patent in France for an effect presently named after Coanda and was described as:
"Deviation of a plan jet of a fluid that penetrates another fluid in the vicinity of a convex wall."
Unfortunately Coanda couldn't obtain funding to continue his research after he wrecked his airplane, and so his contribution to jet propulsion never became widespread. If he had been able to continue his work, France could have had a jet-powered air force before WWII began. Even though he didn't build another jet aircraft, he did make a very important contribution to how the aircraft wings produce lift when he discovered what is now called the Coanda Effect.
A natural question is "how the hell does the wing divert the air down?" When a moving fluid, such as air or water, comes into contact with a curved surface it will try to follow that surface.
Coanda Effect: A moving stream of fluid in contact with a curved surface will tend to follow the curvature of the surface rather than continue traveling in a straight line.
![]() |
![]() |
To perform a simple demonstration of this effect, grab a spoon and find a sink. You can easily demonstrate the Coanda effect for yourself. Conveniently, these are often found together in the kitchen, no need for highly technical lab. Get a small stream of water coming down from the sink, and then place the bottom of the spoon next to the stream. Dangle the spoon next to the stream coming from the tap. I say dangle because you want to hold it loosely enough so it can swing back and forth a bit. It helps to attach a piece of tape at the handle end to act as a hinge. Move the spoon up to the edge of the stream so it barely touches. When you do the water will flow around the bowl of the spoon and off the bottom deflected to the side and the spoon will move into the stream. Spoon is actually being pulled towards the stream of water. Gases behave pretty much like liquids, so when you see the water behaving strangely with the spoon, that's what the air does with the curved paper. Just as water flowing around the spoon's curved surface draws it into the stream, air blown over the curved paper is what causes the lift in that common paper lift demonstration.
What is unusual about the Coanda effect is the fact that the fluid or gas flow is pulled so strongly by a curved surface. With a tap, the water will be projected out at a remarkable distance. The degree to which the water and the curved surface remain attached goes beyond the expected. A concave curve will naturally push the flow, but the fact that a convex one would react so strongly to fluid or gas is unusual.
Same situation apply to the wing. Since air behaves exactly like any fluid, Bernoulli’s principle applies. Any time the wind is blowing or a fan blows air, the pressure of the moving air becomes less than it would be if the air wasn't moving. As an aside, this characteristic plays a huge role in how weather systems work! If you can cause air to move faster on one side of a surface than the other, the pressure on that side of the surface will be less than the pressure on its other side.
One of the most widely used applications of Bernoulli's principle is in the airplane wing. Wings are shaped so that the top side of the wing is curved while the bottom side is relatively flat. In motion, the front edge of the wing hits the air, and some of the air moves downward below the wing, while some moves upward over the top. Since the top of the wing is curved, the air above the wing must move up and down to follow the curve around the wing and stay attached to it (Coanda effect), while the air below the wing moves very little. The air moving on the top of the curved wing must travel farther before it reaches the back of the wing; consequently it must travel faster than the air moving under the wing, to reach the back edge at the more or less same time. The air pressure on the top of the wing is therefore less than that on the bottom of the wing, according to Bernoulli’s principle. The higher pressure air on the bottom of the wing pushes up on the wing with more force than the lower pressure air above the wing pushes down. This result in a net force acting upwards called lift. Lift pushes the wings upwards and keeps the airplane in the air.
Though Bernoulli's principle is a major source of lift in an aircraft wing, Coanda effect plays an even larger role in producing lift.
If the wing is curved, the airflow will follow the curvature of the wing. In order to use this to produce lift, we need to understand something called angle of attack. This gives the angle between the wing and the direction of the air flow, as shown in the following picture.
The angle of attack indicates how tilted the wing is with respect to the oncoming air. In order to produce lift, or downforce acting on the wing, Newton's third law says that there must be equal force acting in the opposite direction. If we can exert a force on the air so that it is directed down, the air will exert an upward force back on the wing. Look at how the Coanda effect directs the airflow for different angles of attack in the diagrams below.
This diagram shows that increasing the angle of attack increases how much the air is deflected downwards. If the angle of attack is too high, the air flow will no longer follow the curve of the wing (Coanda effect is loosing the power). As shown in the bottom of the diagram, this creates a small vacuum just behind the wing. We can say that wing is stalled. As the air rushes in to fill this space, called cavitations’, it causes heavy vibrations on the wing and greatly decreases the efficiency of the wing. For this reason, aircraft wings are generally angled like the middle wing in the diagram. This wing efficiently directs the airflow downward, which in turn pushes up on the wing, producing lift. If you turn this wings on upper picture up side down, you get formula 1 or any wing in use in auto sport. This configuration of the wing, with longer lower part of the wing will produce opposite force, called downforce. But we can apply same rules.
To get around air stream separation problem in airplane wing construction and in Formula 1, and increase the Coanda effect on wings, dual or more element or slot-gap wings are used, these allow for some of the high pressure flow from (in Formula 1 case) the upper surface of the wing to bleed to the lower surface of the next flap energizing the flow. This increases the speed of the flow under the wing, increasing downforce and reducing the boundary flow separation. If you look at a F1 rear wing few years ago on picture above, you can see this concept taken to the extreme, with multi-element wings creating huge amounts of downforce and little air stream separation even on the flaps with extremely high angle of attack.
The Coanda effect has important applications in various high-lift or high downforce devices on aircraft, or in our area of interest, on the racing car wing, where air moving over the wing can be "bent" using flaps over the curved surface of the top of the wing. The bending of the flow results in its acceleration and as a result of Bernoulli's principle pressure is decreased; aerodynamic lift or downforce is increased.
Notice how unlikely is to have a wing in flight with air flow only on one side. The Coanda effect only works in specific conditions where an isolated jet of fluid (or air) flows across a surface, a situation which is usually man-made. You don't find it much in nature. Just so you know, there is no Coanda lift on an airfoil. Coanda effect helps airstream to stay attached to the wing surface, but Bernoulli principle and difference in pressures are the reason why we have a lift or downforce.
Coanda effect is a balancing act between many factors, among them speed of fluids stream, pressure, molecular attraction, and a centrifugal effect if the surface is curved.
Main trouble of the Coanda effect is the airstream becoming turbulent and detaching from the surface, that's how a wing stalls. Pull of surrounding air causes turbulence, drag from the surface and from the ambient air. It's a goal to pull as much as possible ambient air into the airstream, but the drag caused by the difference in velocity between the airstream and the surface is just a loss of energy. If the airstream gets turbulent and stops following the curved surface, there's no more low air pressure, no more thrust.
Since all applications of a Coanda effect involve a fluid object flowing over a solid one, the science behind this effect is known as fluid dynamics. Fluid dynamics represents and study the motion of liquids or gases. Studying this science can lead to many consequential discoveries like the Coanda effect.
The Coanda effect is used on a modern Formula 1 car everywhere sometime to generate downforce, but sometime not for generating downforce directly, but for guiding and conditioning airflow in one place, as a means of maximizing downforce on other. For example, the rear of a modern Formula 1 car is tightly tapered between the rear wheels, like the neck and shoulders of a coke-bottle. By means of the Coanda effect, the air flowing along the flanks of the sidepods adheres to the contours at the rear, and the airflow here is accelerated, creating lower pressure. In itself, this tranverse pressure differential on either side of the car cancels out, and creates no net force. However, the accelerated airflow between the rear wheels and over the top of the diffuser does raise the velocity of the air exiting the diffuser. In addition, bending air away from the rear tires contribute to reducing drag.
The Coanda effect is also used by the bargeboards, aerodynamic appendages typically sited between the trailing edge of the front wheels and the leading edge of the sidepods. Bargeboards are used to guide turbulent air from the front wing wake, away from the vital airflow underneath the car. In addition, the lower trailing edge of a bargeboard creates a vortex which travels down the outer lower edge of the sidepod, acting as a skirt, helping to seal the lower pressure area under the car.
On the end of 2011 exhaust blown diffusers where prohibited by the FIA. Stringent requirements have been placed on the location of the exhaust exit, and engine mapping restrictions are imposed to eliminate off-throttle pumping of the exhaust jet.
In short, these move the exhaust exit to at least 500mm in front of the rear axle line, and 250mm above the reference plane underneath the car. The exhaust exit must also be angled upwards by at least 10 degrees. Hence, it will no longer be possible to blow the exhaust directly between the outer edge of the diffuser and inner face of the rotating rear wheel. Moreover, it will be illegal to place any sprung bodywork in a cone-shaped region, aligned with the exhaust exit, diverging at 3 degrees, and terminating at the rear axle line.
New positioning of the exhaust pipes exits and limitations are shown on picture below. Exits of the pipe can be positioned inside green box with exhaust tailpipe pointed upwards.
But this was not sufficient to eliminate exhaust-blown diffusers. Well, the first thing to note is that it is not possible to point the exhaust exit down at the diffuser in same way as before, this will not necessarily prevent the exhaust jet itself from blowing in that direction. When an exhaust jet exits into a cross-stream of fresh air, the exhaust jet bend with the air stream, effect called "Downwash".
![]() |
Picture from paper published by F. L. Parra and K.Kontis in their 2006 , Aerodynamic effectiveness of the flow of exhaust gases in a generic formula one car configuration |
If the exhaust exit is placed flush in the rearward face of sidepods sweeping downwards at a fairly steep angle, then the free stream airflow could deflect the exhaust jet downwards in direction of the diffuser. The degree to which the jet is deflected is determined by the ratio between the velocity of the jet and the velocity of the cross-stream flow. The smaller the ratio, the more the jet is deflected. This effect is well documented and often termed “jet in cross flow”.
After that, coanda effect will take over and "glue", now energized air stream (mixed with exhaust jet) to the bodywork. Of course, secret is to design this part of the bodywork and bodywork in front of the exhaust exit in the way to optimize this effect and give a proper and exact route for gasses to flow downward in diffuser direction. Effect of diffuser blowing is not as strong as before, but with clever design and optimization you can get few percent more of download. With this set up the exhaust plume is curved downwards by both the shape of the bodywork aft of the tailpipe (coanda) and by the airflow passing over the sidepod (downwash). To learn more about Exhaust Blown Diffuser check my article here.